|
The phylogenetic classification
of the pain pathways is not the only one. The nociceptive pathways can also be
identified simply according to the routes that they follow anatomically. Thus
the archispinothalamic and paleospinothalamic pathways, both of which leave many
collaterals in the reticular formation of the brainstem, are also referred to
as spinal-reticular-thalamic pathways. |
Pain and temperature signals from
the face and the rest of the head are transmitted to the brain by a system that
is similar to but distinct from the one in the spinal cord. This system is composed
of the trigeminal pathways. In these pathways, the cell
bodies of the first sensory neurons are located in the trigeminal ganglion and
in other ganglia associated with cranial nerves VII, IX, and X. The axons of the
trigeminal nerve (cranial nerve V) then enter the pons and descend into the medulla
oblongata, where they synapse with the second neurons in the circuit. As in the
spinal cord, the axons of the second neuron cross the midline and go up the contralateral
side of the body to the thalamus, where they connect into the ventral posterior
medial nucleus of the thalamus, a more medial part of the thalamus than in the
spinothalamic pathway. | | | From
the reception of a pain stimulus in the peripheral nervous system to the perception
of pain in the brain, which generates various
behaviours in response, the pain circuit follows several different, often
redundant pathways. This is not surprising, considering how
important pain is for the body’s integrity. These
multiple nociceptive pathways all start in the same way. A pain signal coming
from the skin, for example, first travels up a sensory nerve fibre composed of
the axons of the T-shaped
sensory neurons located in a spinal
ganglion. These axons then enter the spinal cord, where they immediately divide
and travel a short distance of one or two segments upward and downward in the
spinal cord. They thus form what is called Lissauer’s tract before terminating
in the external part of the dorsal horn. The particular
areas of the dorsal horn where the various fibres (A alpha, A beta, A delta and
C) synapse are not random: they map to a very specific spatial organization. 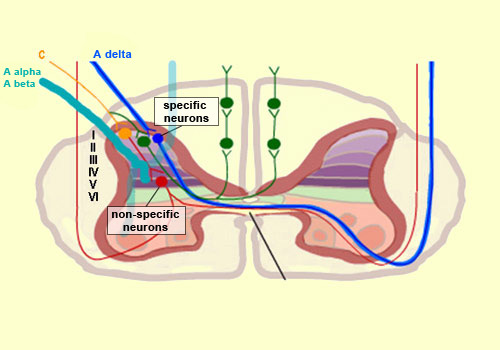
Thus
the large-diameter, myelinated fibres (A alpha for touch and A beta for proprioception),
which ascend directly into the ipsilateral
dorsal column of the spinal cord, nevertheless also send out collateral axons
that penetrate into the deepest layers of the dorsal horn (see box below), which
make direct contact with the deep layers of the ventral horn. There the connections
are made with the motor
neurons that make the withdrawal
reflex possible. Meanwhile, the smaller-diameter
A
delta and C fibres, which transmit pain, synapse on two main types of neurons
that are also located in specific layers of the dorsal root of the spinal cord. The
first type are known as specific nociceptive neurons, and their
cell bodies are located in layers I and II. These neurons receive A delta and
C fibres and hence are activated exclusively by mechanical and/or thermal nociceptive
stimuli applied to the skin. The axons of these specific nociceptive neurons combine
to form the neospinothalamic tract. The
second type are known as non-specific neurons, and their cell
bodies are located in layer V. These neurons respond preferentially but not exclusively
to nociceptive stimuli. They are also known as convergent, polymodal, or wide-dynamic-range
neurons, because they can be activated by fibres carrying non-nociceptive mechanical
stimuli as well as by pain stimuli of tactile, muscular, or visceral origin. These
neurons can also encode the intensity of the peripheral stimulus, by increasing
their firing frequency as this intensity increases. Once this frequency exceeds
a certain threshold, the message becomes nociceptive. The
receiving field on the skin from which these non-specific neurons can be activated
is larger than the field for specific neurons and also displays a sensitivity
gradient: at the centre of the receiving field, all mechanical stimuli produce
a nerve impulse, while at the edge, only nociceptive stimuli do so. The
nerve impulses observed in non-specific neurons can therefore have an early
component, due to the activation of the collaterals of the A alpha and A beta
fibres, and then later
components due to the activation of the A delta and C fibres in response to
more intense stimuli. The fact that fibres of various
diameters and of both visceral and tactile origin converge on these neurons also
explains two distinct phenomena: projected
pain, in which a pain in the internal organs is felt in an area of the skin,
and the segmental
control of pain by the activation of non-nociceptive afferent pathways.
The axons of the non-specific nociceptive
neurons form the paleospinothalamic tract,
which is of earlier evolutionary
origin than the neospinothalamic tract formed by the specific neurons described
earlier. And both of these tracts are newer than the archispinothalamic
tract, which is of very early origin. But all of these tracts are formed
by the axons of the second principal neuron in the nociceptive circuit leading
to the brain (the blue neuron with the blue number 2 in the diagram to the right).
This neuron is located in the dorsal horn of the spinal cord. The expression “principal
neuron” is used here, because the dorsal horn also contains many interneurons
that modulate
the transmission of pain impulses over this circuit. | 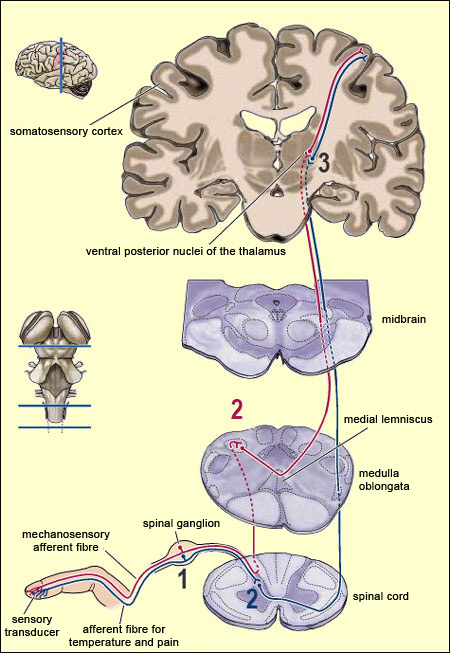
Adapted
from: Neuroscience, 3rd Edition, Purves, Augustine, Fitzpatrick, Katz,
LaMantia, McNamara, Williams, De Boeck, Eds., 2003. | Thus
pain signals are carried over three main extralemniscal pathways (as opposed to
the lemniscal pathway, discussed below) that appeared successively in the course
of evolution: the archispinothalamic tract, the paleospinothalamic tract, and
the neospinothalamic tract. 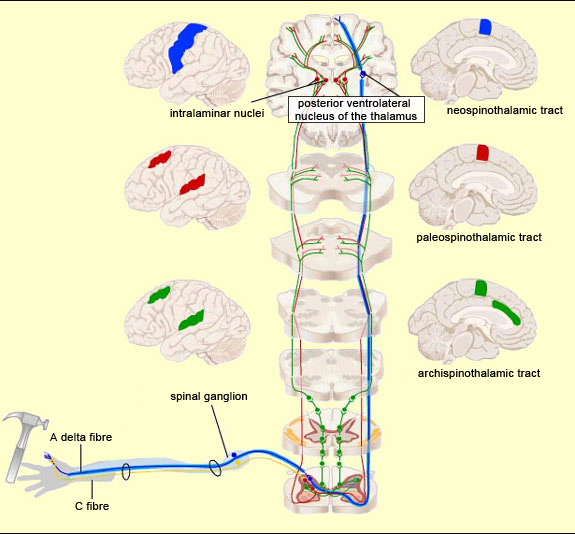
For
an animated version of this figure, see http://neuroscience.uth.tmc.edu/s2/ii7-2.html.
The archispinothalamic
tract or archispinothalamic pathway is the oldest pathway that pain
stimuli can take to reach the brain. It is a multisynaptic, diffuse pathway. The
first places that it reaches in the brain are the reticular
formation and the periaqueductal
grey matter, followed by the intralaminar
nuclei of the thalamus. This tract also sends collaterals to the hypothalamus
and to various nuclei in the limbic
system, thereby contributing to visceral, autonomic, and emotional reactions
to pain.
The pathway
that appeared next in the course of evolution is the paleospinothalamic tract
or paleospinothalamic pathway. (Note that this phylogenetic or evolution-based
classification is not the only possible one—see first sidebar.) The paleospinothalamic
tract is composed of small-diameter
fibres that conduct nerve impulses slowly. Like the archispinothalamic tract,
it has no somatotopic
organization. It projects widely into the reticular formation at all levels
of the brainstem, thus contributing to two important phenomena. The first is the
maintaining of wakefulness in the central nervous system by the ascending reticular
system. The second is the activation of certain nuclei in the brainstem that
constitute the start of the descending
pain-control pathways. The terminations of the
paleospinothalamic pathway also continue into the intralaminar nuclei of the thalamus.
The neurons of these nuclei (which are the third neurons in the afferent nociceptive
pathways, after the neurons in the spinal ganglia and the neurons in the dorsal
horn of the spinal cord) send projections into various cortical regions, including
the frontal
cortex, cingulate cortex, and insular cortex. The
neospinothalamic pathway or neospinothalamic tract,
usually referred to simply as the spinothalamic pathway, is the
most recent nociceptive pathway from an evolutionary standpoint and is found in
higher mammals only. This is the pathway travelled by the rapid
component of pain, which tells the brain about the nature of the painful stimulus
(sting, burn, etc.) and its precise bodily location . This is also the pathway
that transmits the sensation of temperature. The spinothalamic
pathway consists of the axons of the specific neurons of the ventral horn of the
spinal cord. All of these axons decussate, that is, cross over to the contralateral
side of the spinal cord. (They thus differ from the axons in the archispinothalamic
and paleospinothalamic pathways, which make bilateral connections to the brain
structures that they innervate, because some of their collaterals do not decussate
and instead ascend directly on the same side.) Once
they decussate, the axons of the spinothalamic pathway continue their ascent on
the contralateral side, through the anterior lateral part of the spinal cord (which
is why this pathway is also sometimes called the anterolateral or ventrolateral
tract). The axons then enter the medulla oblongata, where they are joined by the
axons of the trigeminal spinal nucleus, which convey pain sensations from the
face (see sidebar), as well as by the medial lemniscal pathway, which is responsible
for the sense of touch. Most of the fibres in the neospinothalamic
tract that come from parts of the body below the neck terminate in the posterior
ventrolateral nucleus of the thalamus. The neurons in this nucleus are the third
in the neospinothalamic pathway and send their axons to the primary somatosensory
cortex.
The dorsal and ventral horns of
the spinal cord contain 10 different layers of grey
matter, known as the Rexed laminae. Just as the Brodmann
areas map the cortex according to the cytoarchitecture (cellular structure) of
each of its parts, the Rexed laminae distinguish 10 different layers in the spinal
cord on the basis of the characteristics of their neurons. The
dorsal horn, where the first connections in the pain pathways are made, contains
laminae I to VI, while the ventral horn, comprising the motor
neurons, contains laminae VII to IX. Lamina X surrounds the central canal. Numerous
studies of the physiology of the spinal cord have confirmed that this division
based on cell types has functional bases as well. | 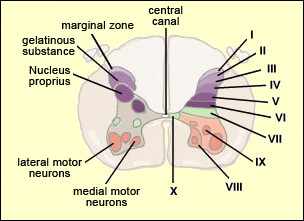 |
| |
|