|
|
|
 |
The
Sense of Self |
 |
|
|
|
Edelman considers primary
consciousness from the perspective of neural darwinism,
a concept that he developed as part of his more general theory
of the selection of neuronal groups. According to this theory,
two different evolutionary processes take place in our brains.
The first occurs while the brain is
still developing,
when a Darwinian competition takes place among populations
of neurons whose connections are only partly constrained
by our genes. The second evolutionary process is a learning
process that has similar mechanisms but that continues in
a more moderate way throughout our lives. Through these two
processes, the brain, which has an overabundance of neuronal
connections at birth, ends up preserving only those that
are sufficiently stimulated in the course of our lives.

|
Edelman’s model
is consistent with Libet’s
experiments showing that even if a behavioural response
occurs very rapidly after the thalamus is stimulated, the
associated conscious experience takes about half a second
to occur. According to Edelman and Tononi, this delay is
due to the long “reentrant loops” that connect
various distant areas in the brain and that must be “closed” for
the conscious impression to arise. |
For Edelman, “reentrant
loops”
differ from what are generally called feedback
loops.
A feedback loop is a simple, well defined
circuit in which the effects come back to act upon the causes—to
correct errors, for example.
In contrast, a reentrant loop develops
in a system that has undergone a form of selection, and this
loop follows multiple parallel pathways where the information
is not determined in advance. Like a feedback loop, however,
a reentrant loop can be either local (confined to a single
neuronal map or neuronal
assembly) or global (distributed across several entire
areas of the brain).

|
Most neurobiological
models of consciousness focus on thalamocortical activity.
But there is an alternative approach that instead assigns
the fundamental role to the subcortical structures.
Swedish neuroscientist Bjorn
Merker, the most ardent champion of this alternative,
points out that “to be conscious is not necessarily
to be self-conscious”. Primary
consciousness (or, in Panksepp’s
terminology, “primary affect”) can be defined
as the ability to integrate sensations from the environment
with one’s personal objectives and emotions of the
moment in order to guide one’s behaviour. And in
Merker’s view, this primary consciousness, whose
contents cannot be expressed verbally, is produced by the
activity of cortical substructures.
For example, Merker suggests that the superior
colliculus, in addition to co-ordinating the
movements of the eyes, to enabling us to pay attention
to things, and to integrating information from various
sensory modalities, may also generate a simulation of
our sensory world—a simulation that corresponds
to what is known as primary consciousness.
The subcortical structures that interest
Merker thus correspond to the upper part of the brainstem,
which runs from the roof
of the midbrain to the base of the diencephalon,
as well as other subcortical structures such as the zona
incerta, the ventral thalamus, and the diffuse
neuromodulation systems of the brainstem.
According to Merker, these subcortical
structures integrate the information that is massively distributed
in the cortex into the sequential, limited-capacity
operating mode of these parts of the brainstem.
And it is precisely this singular operating mode that would
give primary consciousness the properties that we associate
with it and that are necessary for choosing a single, coherent
behaviour.
These subcortical structures would
thus act somewhat like a funnel that channels the myriad
processes occurring in the cortex down into a single conscious
process that is adapted for making decisions in real time
in the real world.
In this context, Merker believes that
the tacit consensus that the cerebral cortex is the “organ
of consciousness” may simply prove to be an error.
Some authors have responded to this idea by stating that
the cerebral cortex can be regarded more precisely as the “organ
of conceptual consciousness and reasoning”, and that
Merker’s observations on primary consciousness and
subcortical structures do nothing to change that.
Nevertheless, Merker’s observations,
such as those regarding children with hydranencephaly,
could have some very concrete implications. Hydranencephaly
is the most severe developmental brain anomaly and is distinguished
from hydrocephalus by the total absence of the cortex. For
Merker, the fact that children with hydranencephaly smile
and laugh in a way that is entirely human and different from
other primates constitutes evidence that the brainstem is
not just a reptilian
relic lying forgotten in
the sub-basements of the brain. In Merker’s view, the
human brainstem is specifically human.
If Merker is correct, this would raise
some serious questions about medical definitions of brain
death that are based on the absence of cortical activity.
At the very least, doctors could no longer assume that hydranencephalic
patients do not need pain medication or anaesthesia during
major surgery.
In the final analysis, these observations
suggest that some subcortical mechanisms play an important
role in what is called the conscious state, and that no description
of conscious phenomena can be complete if it is limited to
the thalamocortical loops alone.
|
|
|
CAN STATES OF CONSCIOUSNESS
BE MAPPED IN THE BRAIN? |
|
Many authors believe that self-awareness,
or the sense of self, is the product
of evolutionary and cultural pressures, and thus not just of
our physical environment but mainly
of the other individuals with whom we communicate. Some authors
therefore believe that human beings’ sense of self may have
co-evolved with our language-related
abilities to engage in abstract, symbolic thinking.
For example, V.S. Ramachandran hypothesizes
that a new set of brain structures evolved in the course of hominization
in order to transform the outputs of the primary sensory areas
into what he calls “metarepresentations”. In other
words, instead of producing simple sensory representations, the
brain began to create “representations of representations” that
ultimately made symbolic thought possible, and this enhanced
form made sensory information easier to manipulate, in particular
for purposes of language.
According to Ramachandran, the interaction
among Wernicke’s area, the inferior parietal lobe (especially
in the right hemisphere), and the anterior
cingulate cortex is fundamental for generating metarepresentations
from sensory representations, thus giving rise to qualia and
to the sense of a “self” that experiences these qualia.
The right hemisphere, because of its
involvement in overall body image, is very important for generating
this feeling of selfhood. But the left hemisphere, which is the
one that specializes in language in the vast majority of people,
seems to be just as essential, in its own way, for what we call
self-awareness.
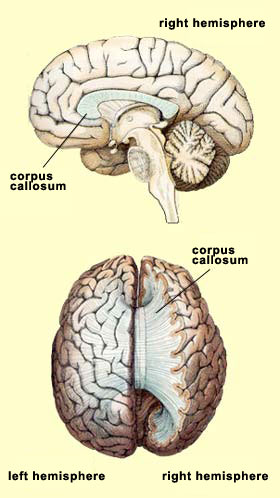
After Hubel, D. (1988). Eye,
Brain, and Vision. |
In any case, that is the conclusion suggested by experiments
that have been conducted on people with split-brain
syndrome. These people have had an operation in
which the corpus callosum (a large bundle of nerve fibres
connecting the two hemispheres of the brain) is completely
sectioned in order to prevent epileptic seizures from propagating
from one hemisphere to the other.
After the operation, these people return to normal life.
In their day-to-day activities, they show practically no
apparent effects of having had their hemispheres separated.
But under experimental conditions in which researchers present
certain stimuli to only one of their two hemispheres, these
people display certain disturbing dissociations.
In fact, it seems that when someone’s brain is divided,
their consciousness is divided as well! |
Michael
Gazzaniga has demonstrated several situations in which
the two cerebral hemispheres of people with split brains come into
conflict (follow the Experiment Module link below for some examples).
In general, if a visual stimulus is sent to a split-brain subject’s
right hemisphere via the left eye, he will state that he has seen
nothing. However, if you let him feel the object with his left hand,
which also is controlled by the right hemisphere, he will then be
able to select the object out of a group, but still won’t
be able to state its name. It is as if there were two persons in
the same individual: one who depends on the left hemisphere and
can speak, and another who depends on the right hemisphere and cannot
speak but can, if questioned correctly, prove that he or she has
perceived something and act accordingly.

When a person with a split brain is placed in a situation
where the two hemispheres come into conflict, she may use
her left
hemisphere’s language capabilities to talk to herself,
sometimes even going so far as to force the right hemisphere
to obey the left hemisphere’s verbal commands. If that
proves impossible, the left hemisphere will often rationalize
or reinterpret the sequence of events so as to re-establish
the impression that the person’s behaviour makes sense.
It was this phenomenon that led Gazzaniga to propose that
there is an “interpreter”, or “narrative
self”, in the left frontal cortex not only of split-brain
patients but also of all human beings. |
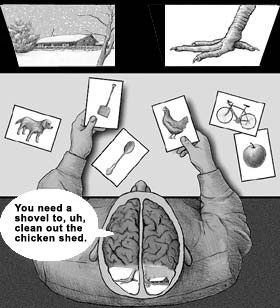
What a patient with a split
brain answers when asked to explain why his left hand chose
the picture of the shovel.
Illustration: Joan M.K. Tycko
|
This interpreter’s role would be to
constantly tell us a coherent story that it constructs from our
actions, emotions, and thoughts. In a sense, this interpreter would
act as the “glue” that lets us assemble all the elements
of our personal history and gives us the impression of being rational
agents.
In fact, according to Gazzaniga, the right
hemisphere may also have its own interpreter. Even though it couldn’t
speak, this observer in the right hemisphere would be better at
understanding irony,
jokes, and other emotional strategies. Each of these two interpreters
would also control the voluntary
movements of the limbs on the opposite side of the body.
So does a person with a split brain have
two consciousnesses? And does a person with a normal brain also
have these two consciousnesses, except that they are so closely
connected that they give us the impression of being just one?
There is no consensus about whether we actually
have an “interpreter”, a sort of inner observer, in
our brains, and some
authors, such as Daniel Dennett, have strongly criticized this
idea. But one thing is certain: a great deal has been written
about split-brain experiments in the past, and they will continue
to generate much discussion in future.
In any
case, while some neurobiological models of consciousness focus
on particular structures in the brain, such as the inferior parietal
lobe, or on one particular hemisphere, other models flatly assert
that consciousness cannot be attributed to any specific
structure in the brain.
In this regard, it should be noted that neuroscientists
have often investigated the neurological bases of consciousness
just as they would those of any other brain function: by analyzing the
contribution of different parts of the brain or different
groups of neurons. But many authors have criticized this conventional
approach, arguing that it is not appropriate for studying so global
a function as consciousness. They point out that damage to almost
any part of the brain has an impact on consciousness, but that
localized lesions, while affecting consciousness, will rarely destroy
it completely. Another fact, emphasized by Gerald M. Edelman and Giulio
Tononi, is that the circuits of the human brain are “massively
degenerate”—that is, highly redundant, so that the
same function can be performed by many different circuits.
Edelman and Tononi thus belong to the school
that believes that the brain as a whole has its role to play in
conscious processes. Their model attempts to explain two characteristics
of consciousness that they regard as fundamental: the fact that
every state of consciousness is an indivisible whole, but that
at any given time, every one of us can choose among countless different
conscious states. In other words, their model attempts to account
for both the unity and the complexity of consciousness.
Like most neurobiologists
who attempt to model consciousness, Edelman and Tononi find
themselves forced to distinguish
at least two major forms of consciousness: primary consciousness
and higher-order consciousness.
Primary consciousness,
which we have in common with many other animals, is the kind that
lets us become aware of the situation that we are in. It relies
on the short-term
memory that makes what Edelman calls the “remembered
present”
possible.
In the Edelman/Tononi model, when a stimulus
is received by the human brain, it forms perceptual maps composed
of neuronal
assemblies whose reciprocal connections are strengthened. The
result is a system of “neuronal maps”,
each of which is responsible for the brain’s various perceptual
capabilities, which result from a selective process that Edelman
calls “neuronal Darwinism” (see first
sidebar). When the brain receives a new stimulus, several of these
maps are activated and send signals to one another. Primary consciousness
arises from the interaction of the various neuronal assemblies
that code for the various properties of an object. This interaction
would occur chiefly through reciprocal connections forming loops between
groups of neurons that are sometimes quite distant from each other
in the brain.
Thus, in this model, primary consciousness
constantly depends on parallel, recursive activity within and between
areas of the thalamus and the cortex. Though Edelman and Tononi
try not to draw too close an association between certain brain
structures and conscious states, they do recognize the undeniable
importance of the thalamocortical loops in the
emergence of consciousness. They accord less importance, however,
to neuronal
synchronization as such than they do to the functional significance
of these loops.
According to this model, the other form of
consciousness—higher-order consciousness,
which emerged during hominization—also depends on such “reentrant
loops” between neuronal assemblies, but in this
case the loops make larger-scale connections in the brain, especially
between the cortical
areas associated with language and those associated with abstract
concepts. The resulting massive increase in the brain’s semantic
capabilities over the course of evolution enabled the concept of
self to emerge, and hence enabled the brain to consider primary
consciousness in light of the past and the future. In other words,
it enabled the emergence of a consciousness of being conscious,
which is the definition of higher-order consciousness.
More specifically, according to Edelman and
Tononi, all of this was made possible by the development of a “dynamic
core”—a vast assembly of neurons that are
constantly being rearranged, but that maintain continuity and
generate an integrated picture from a complex range of possibilities,
which are the two main characteristics of consciousness that this
model seeks to explain. In other words, the dynamic core explains
how consciousness can have only one object at any given time, but
can move very quickly from one object to another.
Just like Llinás and Freeman,
Edelman and Tononi thus emphasize the dynamic aspect of this core,
which here refers not to a stable anatomical structure but rather
to an ephemeral pattern of activity involving neurons from different
parts of the cortex at any given time. And because at any given
time, the dynamic core can involve completely different parts of
the brain, this model avoids the need to identify a particular
area of the brain, or a particular type of neuron, or a particular
frequency of neuronal activity as the source of consciousness.
Instead, this model proposes that a given neuronal activity is
part of a conscious state if, at the time that this state is conscious,
this activity is included in the dynamic core.
This model thus has a great deal in common
with the global
workspace theories of consciousness. It differs from them in
that it does not say that a piece of content becomes conscious
simply because it is made available to the rest of the system.
In other words, the dynamic core model does not equate accessibility
with consciousness the way that global workspace theories do.
Although the neurons in this dynamic system
interact mainly with one another, they also influence and are influenced
by other structures in the brain. Indeed, even though Edelman and
Tononi, unlike some other authors, do not attempt to give certain
areas of the brain a “starring role” in their model
of consciousness, they do identify several brain structures as
playing an important part in it.
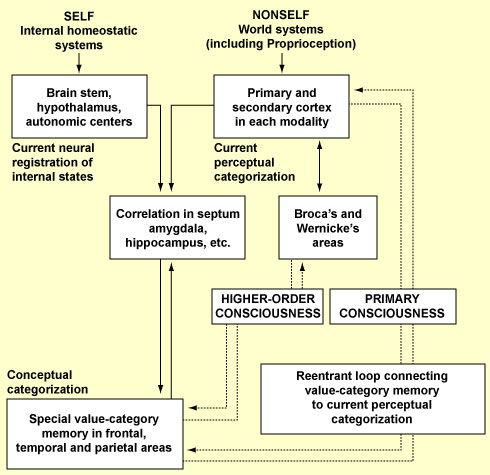
(Source: Susan Blackmore, Consciousness:
An Introduction, after Edelman and Tononi) |
Two of these structures, of course, are the
thalamus and the cortex, which are involved in the formation of
the reentrant loops. But an important role is also played by the
nuclei of the brainstem, whose axons ascend into
vast areas of the brain and diffusely release neurotransmitters
such as norepinephrine,
serotonin, acetylcholine, and dopamine. The activation threshold
of the various neuronal assemblies in the cortex is then determined
by these diffuse
neuromodulatory systems (or, in Edelman’s terminology, “diffuse
ascending value systems”).
Some neurons in these neuromodulating nuclei
will, for example, be
in tonic activity when an individual is awake, and the cessation
of this activity will help the individual to fall asleep. Other
neurons will go into phasic activity when something new or important
for the individual appears in the environment. For example, the
neurons of the locus
coeruleus release bursts of neuromodulators into most
of the structures in the brain; when an individual enters a new
environment, or something unusual happens in his or her surroundings,
the neuromodulator released is norepinephrine.
These neuromodulators can thus modify the
activity of large populations of neurons. They can also change
the likelihood that synapses will be strengthened or weakened in
response to a given neuronal activity. These systems of neuromodulators
are thus extremely well suited for amplifying one type of brain
activity at the expense of another.
Lastly, the ability to choose, from among
numerous inputs, those that have a certain importance in the individual’s
personal history affords a definite adaptive advantage. The hippocampus,
which co-evolved with the cortex and interacts with it to produce episodic
memory, probably plays a key role in this process.
French neuroscientist
Bernard Mazoyer and his colleagues performed a meta-analysis
of several brain-imaging experiments in which the subjects’ brain
activity while they were performing various specified tasks
was compared with their brain activity while they were in
a conscious resting state. When the researchers looked for
commonalities in the images of this resting state from the
various experiments, they discovered a network of brain areas
that are activated jointly during this state. These areas
include the two angular gyri, the left
anterior precuneus, the posterior cingulate
cortex, the left medial frontal cortex,
the anterior cingulate cortex, the medial
frontal sulcus, the left superior sulcus,
and the left inferior frontal cortex.
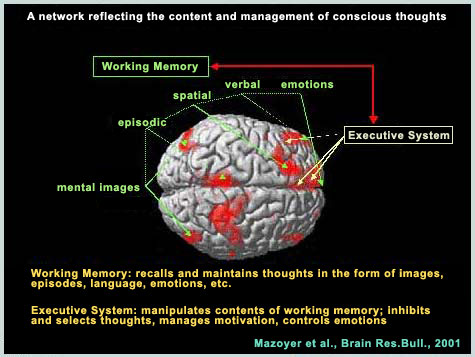
These results suggest that brain activity
during the conscious resting state depends on a large-scale
network of associative parietal and frontal
areas of the cortex. The authors of this study also
suggest that these areas may be organized around two major
functional axes: a parieto-frontal network that
is involved in episodic
working
memory and driven in part by emotions, and an executive
system that is located in the left prefrontal
lobe and supervises this parieto-frontal network.
|
|
|