|
The nervous system’s circuits include mechanisms
by which the efficiency
of all the synapses in a group (and hence the relative weight of each component
in a neural network) can be adjusted continuously. These
mechanisms are also used to place all the neurons in a group of neurons “on
the same wave length.” They can then all be activated simultaneously, which
gives them more impact on each other than on neurons outside the group. In this
way, the brain is believed to form original, temporary configurations of neurons,
known as neuronal assemblies. | |
| The operation of the
circuits of the cortex is often (but not
always) schematized in terms of three processes: information input, synthesis
and comparison, and output and action. Information
input to the nervous system comes through receptors that are sensitive
either to variations in the outside world that are perceived by the sensory organs
or to variations within the body, such as changes in body position. A
receptor may itself be the first neuron in this process (as in the olfactory bulb),
or it may simply be in close contact with the first neuron (as is the case for
the photoreceptors in the retina). Before the nerve fibres emerging from a given
sensory organ reach the primary cortical area where the inputs that they provide
are processed, almost all of them make at least one connection in the brain’s
subcortical centres, of which the main ones are the thalamic nuclei. In fact,
each of the other cortical areas, whether motor or associative, receives nerve
fibres from a thalamic nucleus dedicated to that particular area.
Another important cortical input consists of fibres
from the cortex itself, from either the same hemisphere or the opposite one. These
fibres associate several areas with one another and are therefore called associative
fibres. |  | 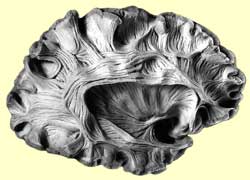 |
Once sensory signals arrive in their primary cortical
area, they quickly diverge into various local circuits responsible for information
processing. All of these cortical microcircuits comprise the same types
of cells distributed in the same six layers of the cortex. The function of a cortical
area is thus determined more by its inputs and outputs than by the intrinsic organization
of its local circuits. The results of the “computations”
performed by these microcircuits ultimately converge at pyramidal neurons whose
axons are the only output pathways from the cortex. A
high proportion of the axons that leave the cortex return to it, either on the
same side of the brain or on the opposite side. But other axons emerging from
the cortex terminate in subcortical centres such as the thalamic nuclei, where
they come into contact with the sensory fibres that send their axons to the cortex.
“Loop”
circuits that return signals to the cortex can thus be formed at this location.
This is a fundamental characteristic of the way that
the brain processes information. At every stage, some of the fibres and connections
loop back to the preceding stage to provide it with feedback that helps to control
it. For instance, such feedback loops enable the brain’s motor control centres
to correct and adjust their signals to the muscles, right up to the moment these
signals are sent. It is feedback loops like these that let you, for example, keep
your balance while walking against sudden gusts of wind. This same phenomenon
of feedback loops is also found in bodily reflexes, such as the leg withdrawal
reflex. 
In this reflex, the sensory neuron that detects
the painful stimulus sends this information to various interneurons, some of which
excite motor neurons, and others of which inhibit them. One interneuron
excites the motor neurons for the flexor muscle in the stimulated leg, causing
this muscle to contract. At the same time, another interneuron inhibits
the motor neurons for the extensor muscle in this same leg, causing this muscle
to relax. The net result: the leg that receives the painful stimulus reflexively
withdraws from it and lifts off the ground. Meanwhile, the opposite leg
will also be influenced by this sensory input, by means of a “cross-reflex.”
In this case, the effects are reversed. On this leg, the motor neurons of the
extensor muscle are excited, while those of the flexor muscle are inhibited. This
opposite leg therefore straightens out and becomes more rigid and stable, to support
the additional weight placed on it when the other leg withdraws from the ground. Short,
simple reflex circuits like these let the body make quick, simple reactions to
protect itself. In contrast, a complex task such as playing a piano involves highly
complex connections, because it requires the pianist to contract and relax so
many different muscles simultaneously. |  | 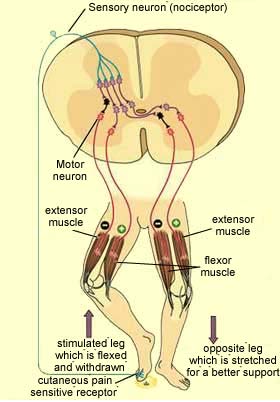 |
The
following diagram gives an idea of the complexity of the cortical circuits that
are known to be involved in processing visual information. | 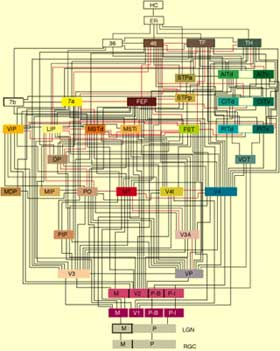 |
Credit: Felleman and Van Essen's
Circuit Diagram of the Macaque Brain as of December 1990 | |  |
Dissection of the left hemisphere, showing the
arcuate fasciculus (the network of long associative fibres that connects the auditory
and motor associative cortexes while going around the end of the fissure of Sylvius.
| 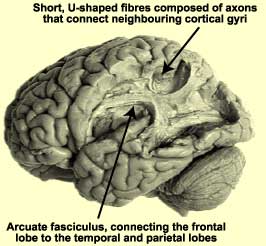 |
Credit: The Digital Anatomist |
| |
|