|
|
|
 |
The
Sense of Self |
 |
|
|
|
 |
 |
 |
 |
 |
 |
Heisenberg’s
uncertainty principle, also known as Heisenberg’s
non-determination principle or Heisenberg’s inequalities,
was presented by German physicist Werner Karl Heisenberg in
the spring of 1927. This principle states that it is impossible
to obtain precise measurements of both the position of a
particle and its momentum at the same time. The more precisely
we determine the one, the less we can know about the other.
In other words, we can either know the particle’s precise
position while accepting great uncertainty as to its momentum,
or vice versa, but we can never know both of these values
precisely.
One way to try to explain this paradox
is to assume that we cannot observe anything unless we expose
it to light—in other words, to photons, which will strike
it and be reflected by it. But on the scale of the infinitely
small, even the tiniest photon that strikes an elementary
particle such as an electron will alter its initial trajectory
or make it change orbital. On this scale, the photon becomes
a veritable projectile that may let us determine the electron’s
position but will simultaneously alter its momentum and trajectory,
so that we cannot know them at the same time as its position
(but see the links below for an update of these explanations)..
|
For the most part, quantum
mechanics describes physical systems in terms of wave functions
that change deterministically over time, in accordance with Schrödinger’s equation.
Developed by Austrian physicist Erwin Schrödinger in 1925,
this fundamental equation of quantum physics describes how
an elementary particle evolves over time.
In this sense, this equation lets us
do the same thing as dynamic equations in classical mechanics:
predict the position and momentum of any system of particles
that evolve deterministically over time. The difference is
that what is known as the quantum wave does not specify the
particles’ position and momentum as such, but rather
the probability that some of the particles will have a given
position and a given momentum when “measurements” are
taken. Quotation marks are used here because the truly strange
thing about quantum mechanics is that it does not provide a
real explanation of what happens during such measurements (see
preceding sidebar).
The idea of the collapse or
the decoherence of the quantum wave is used
to attempt to describe what happens at the time of measurement.
Because, in some way that is not clearly understood, the act
of measurement causes the quantum wave (which usually has a
range of possible positions and velocities) to collapse in
an indeterminate manner into definite values.
It’s a little like playing “heads
or tails” in the dark. The probability of the coin’s
being heads up or tails up when you pull your hand away is
the same, but so long as you have not turned on the light,
you can say that the coin lying immobile under your hand is
in a superposition of states. When you turn
on the light and actually take a “measurement”,
you collapse the superposed states into a single one: heads
or tails.
Though this analogy depicts the mechanism
of this collapse fairly well, it is not described by Schrödinger’s
equation and is the subject of much controversy.
|
|
|
CAN QUANTUM EFFECTS EXPLAIN CONSCIOUSNESS? |
|
There are many different theories that
try to explain consciousness in terms of molecular phenomena.
For example, Flohr’s
hypothesis posits the involvement of NMDA receptor molecules in
our conscious processes. But another large group of theories
draws on the principles of quantum physics to try to explain
consciousness in terms of the infinitely small.
Some of these latter theories are purely
speculative and allude to the strange properties of quantum systems
in a metaphorical sense only. These theories may inspire new
hypotheses that can be tested experimentally, but the theories
themselves do not represent any real scientific progress so long
as they remain nothing but vague analogies.
But other approaches apply current
quantum theory to develop causal models linking specific biological
microstructures to the physiological and psychological mechanisms
of consciousness. For example,
in the 1990s, Beck and Eccles suggested
that the probabilistic nature of the release
of neurotransmitters from the synaptic vesicles into the synaptic
gap was of quantum origin. According to these authors, the
extremely small size of the sites where the exocytosis of the
synaptic vesicles occurs enables quantum uncertainty to play
a role in this process.
Eccles describes structures called “dendrons” composed
of groups of about 100 dendrites of pyramidal neurons in the
cortex. He theorizes that consciousness operates by reciprocally
linking each dendron with its associated unit of mental experience,
or “psychon”. The effect of the psychon on the dendron
would then increase the probability of the synaptic vesicles’ releasing
neurotransmitters into the excitatory synapses of this dendron’s
dendrites.
Eccles thus offers a dualist hypothesis,one
that posits two distinct worlds. In this hypothesis, quantum
physics at the level of the synaptic vesicles plays a role somewhat
like the
role of the pineal gland in the philosophy of Descartes:
the site where the two worlds interact. Such dualism is intriguingly
consistent with Eccles’s religious background: though he
received the Nobel Prize for Medicine in 1963 for his important
discoveries regarding synaptic mechanisms, he was a practicing
Catholic who never disguised his faith in a human soul of divine
origin.
Of all the theories of consciousness that
draw directly on quantum physics, the one with the longest history
was proposed by John
von Neumann in the 1930s, developed further by Eugene
Wigner in the 1960s, and refined a bit further by Henry
Stapp starting in the 1980s.
In his 1955 monograph on the mathematical
bases of quantum physics, von Neumann addressed the thorny issue
of measurement in the context of quantum physics—the famous Heisenberg
uncertainty principle (see sidebar). According to this
principle, the more closely we approach the infinitely small, the
more we realize that what we call reality tends toward a state
that is more potential than real. This suggests that the only thing
that can be considered fixed at this level arises from the act
of observation itself, which in a sense determines one particular
state at the expense of others.
From this came Von Neumann’s idea that
what we call the “observer”of a measurement can be regarded
as the measuring instrument just as much as it can be regarded as
the human brain that takes note of this measurement. Other authors
go even further and state that it is human consciousness that actually
completes the quantum measurement, thus ascribing to this consciousness
a critical role in taking this quantum measurement.
Inspired
by these predecessors, Stapp developed his own interpretation
of this approach. His hypothesis is based on the application
of the uncertainty principle to the ion channels in neurons.
The opening of these channels results in the release of neurotransmitters
into the synaptic gap. And because it is these synapses that
determine our thoughts through the interplay of neuronal
assemblies, Stapp believes that quantum effects in these
ion channels may influence our conscious thoughts. |
|
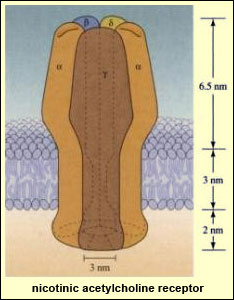
(To
view more diagrams of this receptor, click here.) |
To support
his hypothesis, Stapp notes that for an ion channel with
a diameter of 1 nanometre (10-9 metres), the uncertainty
for momentum is of the order of 1 metre per second, according
to Heisenberg’s principle. In Stapp’s view, these
effects are sufficient to give rise to a superposition
of quantum states (see sidebar) that consciousness
could then reduce to a single classic macroscopic state.
In simpler terms, Stapp believes that quantum waves collapse
when intelligent brains select certain of the available quantum
alternatives to decide
their future behaviour. |
Hence this interpretation of quantum mechanics
is also a theory of consciousness inasmuch as the parts of the brain
that are involved in the collapse of the quantum wave (see sidebar) are the ones that participate directly in consciousness.
Human consciousness would thus have the singular ability to make
the quantum wave collapse—in other words, the ability not
only to describe physical reality, but also to influence it and
hence to influence, in particular, the activity of the brain itself.
Thus Stapp does not so much try to explain
in quantum terms how consciousness may be constituted as he takes
it as given and states that it can influence a quantum phenomenon
such as the collapse of the wave function. And for Stapp, this perspective
can explain two essential things: first, the adaptive
function of human consciousness, which lets us eliminate alternative
realities so that we can choose a more effective course of action,
and second, free
will, which is so precious to us as human beings.
This can be regarded as a somewhat radical
version of what neurobiologists who research attention call “top-down
mechanisms”. In any case, this version goes too far for
the reductionist
materialists, who reject it outright. At the very most, according
to some commentators, it can be seen as having some points of convergence
with the materialist approach known as the “dual-aspect
theory”.
Other theories that postulate quantum effects
as the basis for consciousness find current quantum theory incomplete
and rely on future developments in quantum theory to validate their
intuitions. This is the case, for example, with Penrose
and Hameroff’s model.
Decoherence theory
attempts to resolve the difficult question of why the macroscopic
world is not quantum.
The famous thought experiment known
as Schrödinger’s cat demonstrates
just how difficult this question is. In this thought experiment,
a cat is placed in a closed, opaque box along with a flask
containing a lethal gas. The flask will not release this
poison unless an electron shot from a gun slightly farther
away strikes the upper half of a sensor (and not its lower
half).
Now, the quantum wave of this system
gives the electron just as much chance of striking the upper
half of the sensor as the lower half. The cat’s fate
therefore remains undecided so long as the wave function
has not collapsed (see sidebar) and so long as we do not
know whether the electron has struck the upper or the lower
portion of the sensor.
But when does this happen? When do
things become determined and definite: when the flask breaks?
when the cat inhales the poison gas? or only when it either
lives or dies? And this is where things get really strange,
because if we rely solely on Schrödinger’s equation,
it doesn’t help us at all: according to that equation,
the cat is in a superposition of two states: dead and alive!
In precisely the same way, this equation sees the electron
as a superposition of two trajectories, toward the upper
and bottom halves of the sensor.
Thus physics alone does not seem able
to tell us when things become definite. From this comes the
idea, championed by Stapp, that the wave function may collapse
only at the moment when it interacts with consciousness.
Thus nothing would need to be definite until it was consciously
perceived by an observer. If that is true, then Schrödinger’s
cat would be neither dead nor alive until an observer opened
the box and looked inside.
|
|
|