|
| |
The main steps in synaptic transmission–synthesis,
secretion, binding, and inactivation–are well known for several “classical” neurotransmitters
that have been studied for quite some time, such
as dopamine. On this page we describe these steps using the
example of acetylcholine,
a neurotransmitter that is very common in the central and peripheral
nervous systems as well as in all neuromuscular junctions.
Credit:
Dr. Fred E. Hossler et Dr. Roger C. Wagner, University
of Delaware |
In this photo: the three black
arrows identify synaptic vesicles where neurotransmitters
are stored in the terminal button of an axon, the
letters PC identify two post-synaptic neurons, the
black triangle indicates the thickening of the post-synaptic
neuronal membrane, and the white triangle that of
the pre-synaptic neuronal membrane. |
|
 |
1. Synthesis
“Classical” neurotransmitters
are small molecules that are synthesized locally
in the terminal button of the axon. The precursors
of these molecules are converted into active neurotransmitters
by means of one or more enzymes present in the axon.
To produce acetylcholine, for
example, the enzyme choline acetyltransferase combines
choline with acetyl coenzyme A. Other neurotransmitters,
such as neuropeptides (a
category that includes endogenous opioids) are far
bigger molecules, so they must be synthesized in
the neuron’s cell body, where the organelles
needed to assemble the amino acids are located. |
|
2. Excretion
Most of the synaptic vesicles where neurotransmitters
are stored are attached to cytoskeleton components in the axon’s
terminal button, near the active zones where these vesicles
will fuse with the button’s membrane. When an action
potential reaches the axon’s terminal button, it is accompanied
by a massive influx of calcium ions. These generate a cascade
of reactions that cause the vesicles to detach from the cytoskeleton
components and migrate rapidly to these active zones.
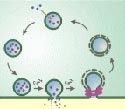 |
 |
In a process called exocytosis,
the vesicles then merge briefly with the terminal button
membrane, release their neurotransmitters into the synapse,
then close up again and retreat inward, ready to be filled
with neurotransmitters again. |
(click
on 6. Neaurotransmitter Release)
3. Binding
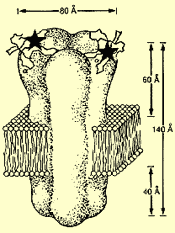 |
 |
If the neurotransmitter released
into the synapse is acetylcholine, for example, it then
binds to acetylcholine-specific receptors on sodium channels
in the post-synaptic neuronal membrane, causing these
channels to open and let sodium ions enter this neuron.
The neuron’s membrane then becomes depolarized,
causing the neuron to become excited. In contrast, when
a neurotransmitter such as GABA or
glycine binds to receptors on chloride channels in the
postsynaptic membrane, it becomes hyperpolarized, and
the neuron is inhibited. |
(click
on 7. Postsynaptic Mechanisms)
4. Inactivation
In this final step, the neurotransmitters
break their bond with the receptors and return into the synaptic
gap, where they must be inactivated for their effects to cease.
Neurotransmitters can be inactivated by one or a combination
of the following processes: 1) they can simply diffuse out
of the synaptic gap; 2) they can be broken down by enzymes
present in the gap, such as acetylcholinesterase, which breaks
acetylcholine down into choline and acetate; 3) they can be
reabsorbed by the terminal button of the presynaptic neuron
(as happens with dopamine,
serotonin, and norepinephrine); 4) they can be removed from
the gap by glial cells known as astrocytes.
(click
on 8. Removal of Neurotransmitter)
| |