|
|
|
 |
Our Evolutionary Inheritance |
 |
|
|
|
The cortex is the thin layer of neurons, about 3
mm thick, that covers the surface of each cerebral hemisphere. In the human brain, the cortex is generally divided into the following three parts that emerged successively in the course of evolution:
- the archicortex (or archaeocortex) developed in association with the olfactory system; it corresponds
to the dentate gyrus
and hippocampus in mammals and does not yet have the six layers of the neocortex;
- the paleocortex is also associated with the olfactory system and is not stratified into layers; it corresponds to the piriform cortex
and the parahippocampal gyrus;
- the neocortex (or isocortex) is the part of the cortex
that appeared most recently and constitutes by far the largest
portion of the cortex in primates; it is stratified into six
distinct layers of neurons. |
A larger cortex obviously contains a larger number of neurons. But what increases the most in mammals is the number of connections between these neurons. As a corollary, the proportion of white matter in the brain (and hence of the axons that make the connections) grows steadily as one proceeds from rats to human beings. |
Over the course of evolution, the cerebral cortex has grown considerably in surface area but very little in thickness. Thus, in humans, the cortex is only 15% thicker than in macaque monkeys, but has at least 10 times more surface area. The difference between the human cortex and the mouse cortex is even larger: the human cortex is only twice as thick, but has about 1 000 times the surface area! |
The brain does not have just one single function on which evolution might operate. The brain is rather a collection of systems (or, as some would call them, modules), each controlling different functions.
In the realm of emotions, for example, we know a fair amount about the various systems where feelings of fear, anger and disgust originate.
Consequently, evolution tends to act on these systems individually rather than on the brain as a whole. For example, evolution may have favoured growth in the overall size of the primate brain, but its influence is seen mainly in specific systems. |
In vertebrates, cephalization generally increases
as one proceeds from the oldest taxonomic groups (fish) to
the most recent ones (mammals). Among invertebrates, however,
the process appears to be associated more with general body
shape, diversity of sensory organs, habits, and variety of
behaviours than with the group's place in the phylogenetic
tree.
|
|
|
THE EVOLUTIONARY LAYERS OF THE HUMAN BRAIN |
|
The model of the triune
brain proposed by MacLean in 1970 is a useful piece of shorthand
for the complex evolutionary history of the human brain. But the
brain's combination of reptilian, paleomammalian and neomammalian
structures is far more intricate than a mere set of nested Russian
dolls.
Ever since the first mammals appeared more than 200 million years ago, the cerebral
cortex has assumed greater and greater importance compared with the brain's other,
older structures. Because these structures had proven their effectiveness for
meeting certain fundamental needs, there was no reason for them to disappear.
Instead, evolution favoured a process of building expansions and additions, rather
than rebuilding everything from the bottom up.
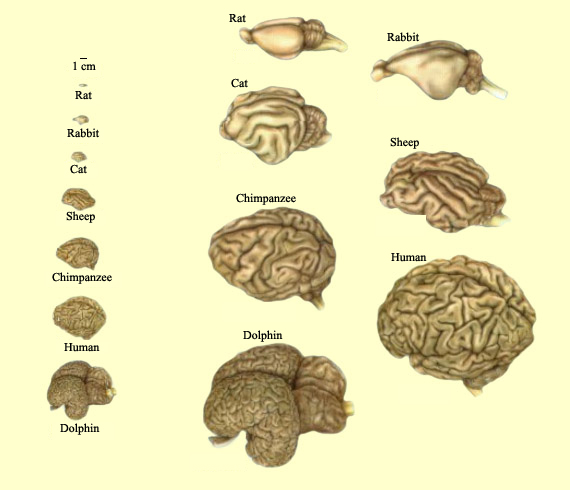
The brains of various species
of mammals
(Left: all on the same scale; Right: enlarged, on various scales)
This expansion of the surface of the neocortex (also
known as the isocortex) is more apparent in predatory mammals than
in herbivorous ones. Catching prey may be difficult, but a successful
hunt provides a far more nutritious meal than any plant. To hunt
successfully requires a highly evolved sensorimotor system. Mammals
with a large neocortex thus have an advantage, because that is
where the sensory and motor regions are located.
Scientists have also observed that the size of the neocortex has
increased tremendously in primates, from the smallest monkeys,
such as lemurs, on to the great apes and human beings. Many scientists
believe that this growth in the primates’
neocortex reflects the growing complexity of their social lives.
Indeed, the ability to predict the behaviour of other individuals
within a group seems to have conferred a large evolutionary advantage.
Thus evolution would have favoured the growth of certain parts
of the cortex that are responsible for social skills such as language,
because they improved this ability.
Another point on which everyone
agrees is that the increase in the folds of the cortex has been
a major factor in the evolution of the brain. These folds, by enabling
a larger cortical surface area to fit inside the cranial vault,
allow for a better organization of complex behaviours.
It is still common to
hear other animals discussed as if they were some inferior
form of human beings —
as if there were some kind of natural ladder on which human
beings obviously occupied the top rung.
But that is not what we actually see in nature. Every evolutionary
line has developed independently, and rats, for example, are
perfectly adapted to their environment. They are not in the
process of extinction, and they live in perfect harmony with
their surroundings. The same could be said for most of the
species that populate the Earth, even though their brains are
far smaller than ours.
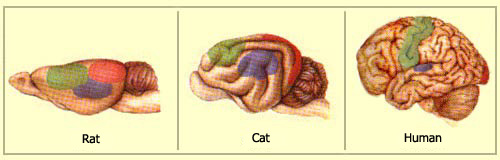
Growth in size and importance
of the associative areas of the brain, from rats to cats
to humans. Green = sensorimotor area, red = visual area,
blue = auditory area.
Consequently, success, from an evolutionary standpoint, does
not depend on brain size. We also know that larger brains
have not replaced smaller ones, but have simply added to
them, thus expanding the existing distribution of brain
sizes.
Countless perfectly adapted small brains can still be found
in animals today. The larger brains that developed later
on are simply the result of evolution's intrinsic tendency
to try out all the possibilities. Thus the growing complexity
observed everywhere in nature is not the “objective” of
evolution, but rather a side effect of its endless experiments
over thousands and thousands of years.
|
Is there a relationship between the size of an animal's brain
and some kind of “behavioural complexity”? Not really.
Elephants and whales have brains 4 to 5 times the size of a human
being's, yet their behaviour is generally agreed to be less complex
than ours.
To identify a meaningful relationship between brain size and behavioural complexity,
we must consider an animal's brain size in proportion to its body size, because,
to cite just one example, an animal with a larger body surface will automatically
need larger sensory areas in its brain. Once we weight brain size for body size,
we do in fact observe a relationship between the size of the brain and the complexity
of behaviour.
But the differences in the evolutionary development of the various parts of the
brain have an even greater effect on behaviour than brain size does. For instance,
the cerebellum is a part of the brain involved in co-ordinating muscle movements,
and its weight as a percentage of the brain's total weight remains remarkably
constant in all mammals. In contrast, the percentage for the neocortex varies
greatly from one species to another. In fish and amphibian brains, there is no
neocortex. In shrews, the neocortex accounts for 20% of the brain's weight, and
in humans, it accounts for 80%!
It was during the transition from primates to human beings that the neocortex
underwent the greatest development. And among all the regions of the neocortex,
it was definitely the prefrontal
cortex that expanded the most in human beings.
Evolutionary biologists
make a distinction between homologous and analogous anatomical
structures.
Two structures are said to be homologous if
they have a common embryonic and phylogenetic origin. For example,
a human being's forearm, a bird's wing, and a dolphin's pectoral
fin are all composed of the same bones, inherited from a common
distant ancestor, and are therefore considered homologous structures.
The very existence of homologous structures in the animal kingdom
is regarded as one
of the strongest proofs of evolution.
In contrast, analogous structures are
structures that fulfil the same functions
in different species but that are derived from two completely
different evolutionary lines. An insect's wings and a bird's
wings offer a good example. Both are used to fly, but taxonomically,
they have nothing in common with each other. Analogous structures
are the product of “"convergent evolution";
they represent differing solutions that various organisms developed
independently to cope with the same constraints in their environments.
|
|
|