|

Science
Starting To Identify the Molecular Bases of the Sense of Touch
Many toxins can affect neuromuscular
junctions and their nicotinic receptors. Some toxins, such as the botulin toxin,
act on the presynaptic side of the junction. They prevent it from releasing acetylcholine
and thus produce an effect of muscle weakness or paralysis. Other toxins,
however, act directly on the nicotinic receptor. They occupy the acetylcholine-binding
site but do not cause the channel to open. Hence the acetylcholine that has been
released into the synaptic gap cannot bind to the receptors, so the muscle cannot
contract. This is how curare works (the poison in which Amazon Indians dip their
arrows): it kills by paralyzing the muscles of the diaphragm. This same mechanism
is at work with bungarotoxin, a type of snake venom. Still other toxic
substances lodge in the central channel of the nicotinic receptor, thus blocking
the passage of ions. This is what happens with procaine, lidocaine, and benzocaine,
all of which are molecules used in local anesthesia, as well as with tetrodotoxin,
a toxin that is found in the livers of certain fish and that can cause death within
a few hours of ingestion. | | |
NICOTINIC ACETYLCHOLINE RECEPTORS | | To make a muscle contract,
the acetylcholine produced
in the presynaptic neuron of the neuromuscular
junction must bind to the nicotinic receptors on the postsynaptic side. Each
of these receptors consists of 5
subunits that form a pentagonal structure around a central channel.
(click on 3. Ions and Ion Channels)
The
nicotinic receptor is a channel (or ionotropic)
receptor: the same protein both forms the transmembrane channel and binds the
acetylcholine or one of its agonists,
such as nicotine.
When one of these substances binds to the receptor, the channel opens, allowing
many sodium ions to enter the post-synaptic cell and a few potassium ions to leave
it, thus depolarizing it. There are two types of nicotinic receptors: N1,
found in the autonomic nervous system, and N2, found at neuromuscular junctions.
These receptors belong to a large family of channel receptors which include those
for glycine, glutamate
(NMDA and AMPA) and GABA (GABA-A
receptors ). | 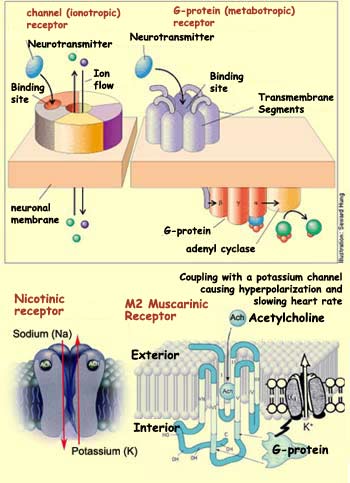
|
In addition to nicotinic receptors, there is another
family of acetylcholine receptors, the muscarinic receptors. They belong to another
large class of receptors called G-protein (or
metabotropic) receptors. The receptors in this class (which includes dopamine
receptors, for example), are totally separate from the ion channels. They exert
their effects on these channels via a protein located on the cytoplasmic side
of the cell, known as G-protein because it binds GTP. When a neurotransmitter
binds to and activates a G-protein receptor, this receptor in turn activates this
G-protein, which controls the opening of the physically separate ion channels,
either directly or indirectly (via a second messenger). G-protein receptors therefore
act more slowly than nicotinic receptors, where everything is centralized on the
same protein complex. Also, whereas nicotinic receptors
are composed of five distinct peptides, the seven transmembrane domains of muscarinic
receptors all come from a single protein that snakes its way back and forth across
the membrane. There are at least five different types of muscarinic receptors,
all of which can be activated by muscarine, a molecule produced by a mushroom.
M1 and M3 receptors, for example, activate phospholipase C, a second messenger
that brings about depolarization by opening calcium channels while reducing the
flow of potassium. In the brain, M1 receptors are found in the cortex and the
central grey nuclei, while M3 receptors are found in the cerebellum. Both types
of receptors are also involved in exocrine gland secretions. The action
mechanism for type M2 receptors is different. These receptors are coupled with
a G-protein that inhibits adenyl cyclase. The reduction in the activity of this
enzyme reduces the amount of the second messenger cyclical AMP, allowing the potassium
channels to open and hyperpolarizing the cell. M2 receptors are also found not
only in the central
nervous system (cerebellum, central grey nuclei, and brainstem) but also in
the heart. | |